by Ashutosh Jogalekar
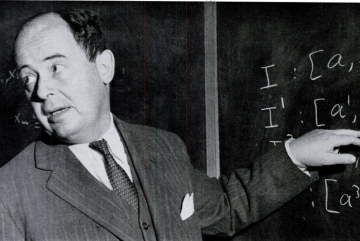
During a wartime visit to England in early 1943, John von Neumann wrote a letter to his fellow mathematician Oswald Veblen at the Institute for Advanced Study in Princeton, saying:
“I think I have learned a great deal of experimental physics here, particularly of the gas dynamical variety, and that I shall return a better and impurer man. I have also developed an obscene interest in computational techniques…”
This seemingly mundane communication was to foreshadow a decisive effect on the development of two overwhelmingly important aspects of 20th and 21st century technology – the development of computing and the development of nuclear weapons.
Johnny von Neumann was the multifaceted intellectual diamond of the 20th century. He contributed so many seminal ideas to so many fields so quickly that it would be impossible for any one person to summarize, let alone understand them. He may have been the last universalist in mathematics, having almost complete command of both pure and applied mathematics. But he didn’t stop there. After making fundamental contributions to operator algebra, set theory and the foundations of mathematics, he revolutionized at least two different and disparate fields – economics and computer science – and made contributions to a dozen others, each of which would have been important enough to enshrine his name in scientific history.
But at the end of his relatively short life which was cut down cruelly by cancer, von Neumann had acquired another identity – that of an American patriot who had done more than almost anyone else to make sure that his country was well-defended and ahead of the Soviet Union in the rapidly heating Cold War. Like most other contributions of this sort, this one had a distinctly Faustian gleam to it, bringing both glory and woe to humanity’s experiments in self-elevation and self-destruction.
The origins of Johnny’s far-reaching accomplishments lay in the Manhattan Project. But this fact alone is curious: Von Neumann was never part of the regular cast of characters at Los Alamos which included Robert Oppenheimer, Hans Bethe, Edward Teller, Richard Feynman, Enrico Fermi and other world-renowned scientists; he features as a relatively minor player in most standard histories of the project. He visited as a consultant a few times a year. And yet in some sense, his contribution equalled or even exceeded in the long-term the contributions made by his fellow scientists. This discrepancy between his role in the Manhattan Project and the importance of his work speaks to both his awesome intellect and, more interestingly, to a core element in the history and philosophy of science in which ideas and technologies unexpectedly intersect and piggyback on each other. To understand this more fully, it’s important to understand von Neumann’s origins.
From Budapest to Los Alamos via Princeton
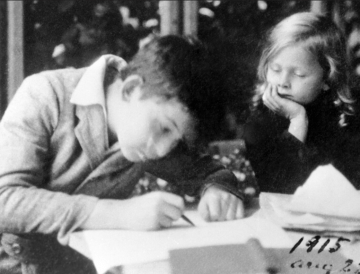
Von Neumann was the quintessential product of turn-of-the-century Jewish affluence and intellectual achievement in the Austro-Hungarian empire. Born in 1903 to a wealthy banker and his wife in Budapest, Johnny was one of history’s great child prodigies, speaking half a dozen languages and learning calculus by the time he was barely past the first decade of his life. He had an amazing photographic memory and an intense interest in history that stayed with him all his life and dazzled his friends and colleagues; as the story goes, by the time he was eight he had read and annotated all forty-three volumes of a comprehensive world history written by the German historian Wilhelm Oncken, and he used to stun his parents’ friends by reciting entire pages from the phone directory as a child. As he grew up he collected around himself some of the great Hungarian minds of the 20th century – Eugene Wigner, Leo Szilard, Edward Teller. Later all of them became émigrés to the United States, and their superior intelligence led others to joke that they were Martians who had learnt to perfectly mimic human beings. Toward the end of his life, Eugene Wigner who won a Nobel Prize for his work on nuclear structure was asked why a tiny country like Hungary produced so many scientific geniuses. Wigner said that Hungary had produced only one genius – Johnny von Neumann.
After getting two degrees in Zurich and Budapest, one in mathematics and one in chemical engineering – the latter to to please his father who, like many Jewish fathers, worried that his son might not be able to get a job in spite of his great intellect but due to his Jewish background – von Neumann became an assistant to David Hilbert, one of the 20th century’s greatest mathematicians. While his early forays were in set theory and operator algebra, in a short time he left a blazing trail of contributions whose depth and breadth would be unequalled in the annals of 20th century mathematics and science. By his 30th birthday, he had solved Hilbert’s fifth problem for compact groups, proved the mean ergodic theorem, provided a mathematical foundation for quantum mechanics, proved the minimax theorem, started laying the foundation for game theory in economics and done important work in the foundations of mathematics.
He had already become acutely prescient about the deteriorating political situation in Europe. After Hitler became chancellor of Germany in 1933, Johnny started making trips to the United States where he had been invited to lecture at the Institute for Advanced Study. The institute had been set up as a kind of pure thinkers’ heaven by the renowned educator Abraham Flexner, and there were few fields more suited to pure thought than mathematics, philosophy and theoretical physics. Flexner went to great lengths to snag the greatest fish of them all – Albert Einstein. By 1933 Einstein had already been the target of virulent anti-Semitic attacks and was looking for a new home. After visiting a few universities in the United States and England, Flexner lured him to Princeton with the promise of a very generous salary and complete freedom to explore his interests without any administrative or teaching responsibilities. But even by 1933, when Einstein was fifty-four, the twenty-nine-year-old von Neumann was considered important enough to be made one of the first professors at the institute, along with Einstein, Hermann Weyl and Oswald Veblen, a mathematician who was as adept at hacking away at the brush in the Princeton woods as he was at problems in geometry and topology. Veblen was to become an important mentor to Johnny. When the institute’s plans to hire Weyl fell through, Johnny was hired as a permanent faculty member.
Ever since he moved to the United States, Johnny showed a great love for his adopted country. His home was the scene of weekly raucous parties, and he loved fast cars, expensive suits and hobnobbing with the rich and famous. His friend Eugene Wigner who had shipped from Hungary as a joint appointment with von Neumann recalled how Johnny was refreshed by the new world and by the youthful enthusiasm he saw there which rejected the reactionary and orthodox ideas of old world Europe, a motivation not uncommon among American émigrés. As Europe crumbled in the face of fascism in the next few years, Johnny was joined by European scientists like Bethe, Fermi and Weisskopf who became household names, started great schools of science and catapulted America into the front ranks of science and technology, a position that it continues to hold in no small part due to such immigrants.
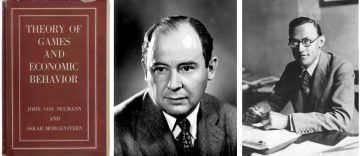
Because of his intense interest and background in history, Johnny could already see in 1935 that there would be some kind of war between Germany and other countries. By 1937 he had started expressing interest in the more applied parts of mathematics. His work on the minimax theorem and the foundations of quantum mechanics was already dazzling, especially for a pure mathematician, and in Princeton he had struck up a friendship with another Austrian emigre, the economist Oskar Morgenstern. During the next few years he would embark on the writing “The Theory of Games and Economic Behavior” with Morgenstern which is now considered the foundation of game theory.
What accounted for Johnny’s forays into applied mathematics and away from pure mathematics? One reason was certainly his capacious mind that roamed over diverse domains out of sheer burning intellectual curiosity, but I also personally feel that it was partly a disillusionment with the foundations of mathematics, a disillusionment that was made acutely clear by the work of his friend and institute colleague Kurt Gödel who in 1932 had struck a blow at the foundations of mathematics through his famous incompleteness theorems. Johnny had extended Gödel’s first theorem in short order, only to find that Gödel had already gotten there. Later he did what he could to ensure Gödel’s appointment at the institute; the two friends now lie only a few feet from each other. Gödel’s work might have convinced Johnny that making deep contributions to pure mathematics was perhaps not his forte. Fortunately for him, the escalating situation in Europe brought a new opportunity that would make full use of his intellectual powers.
Shaped charges and the Aberdeen Proving Ground
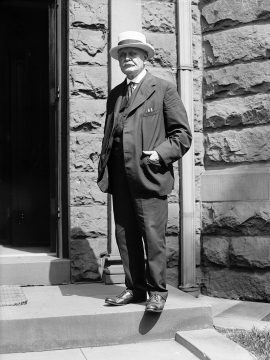
In 1888, an American chemist working for the navy named Charles Munroe discovered something quite wonderful and intriguing. Monroe found that when he detonated a block of explosive with the company’s name engraved on it with raised letters near a metal plate, the raised letters somehow got “transferred” into the metal plate. What was happening was that the explosive blast from the block took the shape of the letters – and stayed that way for some time. Later Munroe demonstrated this effect even more convincingly with a metal safe. As he described it in a 1900 ‘Popular Science’ article,
“Among the experiments made … was one upon a safe twenty-nine inches cube, with walls four inches and three quarters thick, made up of plates of iron and steel … When a hollow charge of dynamite nine pounds and a half in weight and untamped was detonated on it, a hole three inches in diameter was blown clear through the wall … The hollow cartridge was made by tying the sticks of dynamite around a tin can, the open mouth of the latter being placed downward.”
Thus was born the principle of the Munroe Effect and the shaped charge, a phenomenon which still has a little bit of a whiff of magic to it. The shaped charge essentially enables a precisely geometrically-shaped explosive to ephemerally create a small pocket of air that is packed with deadly force – hell in a puff of air. When this puff hits a target, even a metal safe cannot help but be cast in its shadow. Shaped charges soon started seeing use in many peaceful applications of explosives to create controlled, precisely shaped, powerful cavities in materials like rocks and metals. By the time the 1930s came along, scientists in many countries had gotten interested in them. A particularly important contribution was made by R. W. Wood, a Johns Hopkins physicist whose initial interest stemmed from morbid accidental deaths by shaped charges.
An illuminating history of shaped charges tells the story of how the technology gradually percolated from America to Britain and back to America. But there was an ominous detour in Germany. An Austrian physicist named Rudolf Thomanek had become interested in the British and American work and was trying to develop an anti-tank gun. By 1932, he had become convinced that the Austrian authorities were “halfwits” who were uninterested – why not try to sell the concept to the much more enthusiastic German authorities? In fact, why not go straight to the top? By the end of 1935, Thomanek had pulled enough strings to find himself presenting the idea to an audience consisting of Hitler, Himmler, Goering and the General Staff of the Third Reich’s armed forces. Contrary to his later, well-deserved image as a strategist who routinely came up with unworkable and outlandish schemes, Hitler was extremely detail-oriented and well-versed when it came to weaponry. Thomanek had put a dented plate on Hitler’s desk.
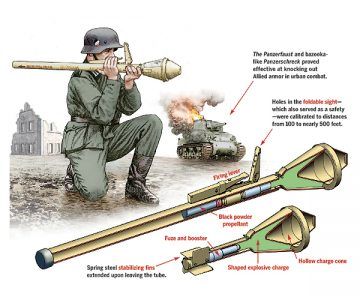
“I am in a hurry and will express my opinion immediately”, Hitler said. “This proposal could be the solution I have always wanted to give the individual soldier a weapon to defeat tanks. Maybe the same principle could be used in bombs and torpedoes.”
The Führer had grasped the significance of the new idea immediately. So did scientists, engineers and politicians in Britain, the Soviet Union and the United States. In the United States the shaped charge saw incarnation as the fabled anti-tank weapon the Bazooka, a weapon whose detailed workings were kept secret until 1945 when the core principle was revealed by a reprint of Munroe’s 1900 Popular Science article. The point of this slight digression is to indicate that by 1937, shaped charges were a well-studied form of anti-tank weaponry. They were also ideally suited to von Neumann’s particular skill set, and it was at the Aberdeen Proving Ground that they found fertile landfall.
The Aberdeen Proving ground in Maryland had been set up during World War 1 for ballistics research. Ballistics research was a clear priority for any army utilizing artillery. The main area of research for ballistics specialists was firing tables that indicated the velocity and the angle at which to fire a projectile to hit both stationary and moving targets. In 1918, Oswald Veblen had been hired to oversee scientific work at the site, and among others had recruited von Neumann’s fellow mathematical prodigy Norbert Wiener to work with him. By the late 1930s, Aberdeen was in a good position to contribute to the war effort and Veblen invited von Neumann to join him. The newly naturalized Johnny was eager enough that he applied to take an exam as a lieutenant in the army reserve in 1939. Amusingly, even though he passed the exam, he was turned down because of an age cutoff. Senator William Smathers from New Jersey expressed his perplexed reaction to FDR’s Secretary of War, Harry Woodring: “Mr. von Neumann is internationally known as a mathematician, and I cannot understand how a man with so much potential value to the American armed forces should be turned down for a technicality.”
The reason Smathers and Veblen considered Johnny so valuable to a potential war effort was simple. Although von Neumann had no training in war-related work, his singular skill as a universalist mathematician would be invaluable in analyzing the nonlinear phenomena involved in shaped charge detonation. Crudely speaking, nonlinear phenomena deal with cases in which a given stimulus produces a disproportionate response. Most complex phenomena in the real world of interest to scientists are nonlinear, and in that sense the name ‘nonlinear’ is a misnomer: As von Neumann’s best friend Stanislaw Ulam who had escaped Poland before the Nazis invaded it once said, “Using a term like nonlinear science is like referring to the bulk of zoology as the study of non-elephant animals.” Nonlinear phenomena required mathematical manipulation at the highest level because of the complexity of the equations which usually involved partial differential equations in multiple dimensions. Both the versatility and speed of von Neumann’s mind made him an ideal candidate for analyzing these. As we will see later, Johnny’s interest in nonlinear equations was to have an unexpected and revolutionary impact on modern technology.
As the United States entered World War 2 after Pearl Harbor, most European émigré scientists wanted to do their part to help the war effort. Johnny’s point of departure into what became his most important contribution arose from Hans Bethe and Edward Teller’s work on shock waves. In 1941, Teller and Bethe had analyzed how the change in conditions around a shock wave, both in its front and back, can be analyzed in terms of characteristics like the medium’s temperature, pressure etc. To a simplifying approximation, Teller and Bethe found that the conditions at the front of the developing wave can be represented accurately by knowing the conditions at the back of the wave. Johnny was aware of this work, and in May 1941 wrote a letter to Bethe in which he asked the physicist if he and Teller could extend their work to air at 25,000 degrees centigrade. The scientists were finding out, interestingly, that the so-called equations of state for materials that relate pressure, temperature and density to each other were actually simplified at higher temperatures compared to lower ones because of a kind of equalizing behavior of different materials at those temperatures. Johnny’s main contribution had been to figure out that the behavior of the detonation of a shaped charge could be interpreted as the propagation of a shock wave followed by chemical reaction of the materials in the medium. We thus know that even before Pearl Harbor, Johnny was already making himself an expert in the behavior of shock waves at high temperatures.
From shaped charges to implosion
Curiously, von Neumann wasn’t involved in the early developments leading to the Manhattan Project, perhaps he already had much on his plate at that point. Not only was he consulting for multiple departments of the army and navy, but he was also making trips to England as a consultant. The early steps leading to the bomb project were all taken by physicists like Leo Szilard, Edward Teller, Robert Oppenheimer and Enrico Fermi. After the news of fission came to the United States in 1939, Szilard convinced his friend Einstein to write a famous letter to FDR impressing on him the urgency of starting research into uranium fission. By the summer of 1942, Oppenheimer had been picked by Leslie Groves, the head of the project, to oversee a preliminary theoretical effort into a fission bomb. At Berkeley that summer, Oppenheimer, Bethe, Teller and others convinced themselves that theoretically, a simple fission bomb would be possible. By the end of 1942, Enrico Fermi and his team had done a proof-of-concept experiment and built the world’s first nuclear reactor at the University of Chicago. With Fermi’s experiments the Manhattan Project kicked into high gear, and by March 1943 an isolated site set among the majestic mesas of Los Alamos in New Mexico had been selected for the top-secret project.
As has been well documented, the basic principle of building a fission bomb was clear by 1943. You had to fire two subcritical pieces of uranium into one another, and once the critical mass had been exceeded there would be an explosion. It was also understood that this process needed to be exceptionally fast to prevent pre-detonation or a fizzle which would give suboptimal yield. The main challenge was always considered the separation of uranium isotopes and the production of plutonium, a novel material which would be even more fissile. By the time the scientists settled in at Los Alamos, Oppenheimer’s close collaborator and confidant Robert Serber had given a set of lectures summarizing the state of knowledge.
It was then that a physicist named Seth Neddermeyer came up with an entirely novel approach to detonating a bomb – an approach called implosion. Instead of crashing two pieces of fissile material together, implosion called for bringing together those pieces inward, preferably in three dimensions to maximize efficiency. It says something about how novel and speculative this approach was that Neddermeyer’s idea met with fierce opposition from the likes of scientists like Bethe, Fermi and Feynman, men who weren’t known for a deficiency of new ideas. Deke Parsons, a naval physicist who was called in to help with ordnance, derisively called it the “beer can experiment”: the challenge was to symmetrically implode or squeeze a beer can from all sides without having the beer squirt out. But Oppenheimer was more prescient and he asked Neddermeyer to conduct preliminary experiments. Stymied by complex three dimensions, Neddermeyer started working with cylinders in two dimensions. By September 1943, the work was trudging along and Neddermeyer was not a particularly harmonious individual to work with, sticking to his ideas and seldom entertaining others. More importantly, what had been already suspected by Oppenheimer’s team in 1942 now became clear – that when it came to building a bomb, understanding the hydrodynamics or flow of shock waves was as important as understanding the nuclear physics and flow of radiation and particles like neutrons. Enter Johnny von Neumann.
Oppenheimer had written a letter to von Neumann in July 1943, and the contents of that letter make clear both the high regard in which he held Johnny and the difficulties he was facing:
“We are in what can only be described as a desperate need of your help. We have a good many theoretical people working here, but I think that if your usual shrewdness is a guide to you about the probable nature of our problems you will see why even this staff is in some respects critically inadequate…I would like you to come as a permanent, and let me assure you, honored member of our staff. A visit will give you a better idea of this somewhat Buck Rogers project than any amount of correspondence.”
At this point it’s worth noting von Neumann’s salient qualities that were considered to be helpful in such a project. I have noted these qualities in detail before in an essay about his major contributions to computing. In a nutshell, while Johnny’s mind was as original as anyone else’s, his main strength was being able to jump ten steps ahead of everyone with his lightning fast mind, look at the big picture and connect disparate ideas together. This enabled him, in his friend Edward Teller’s words, “to identify solutions where most people didn’t even notice the problems.” All this not only made von Neumann a supremely reliable mind to seek advice from but lent his words enormous prestige. Johnny agreed to visit Los Alamos, not as a permanent member but as a consultant in September 1943. When not at Los Alamos, he would work out of the office of the National Academy of Sciences in Washington, D.C.
What Johnny essentially did in his critical visit was anoint implosion with his blessings. He confirmed that the best way to achieve implosion would be to spherically place shaped charges around a uranium or plutonium core; the Munroe Effect operating in three dimensions would then squeeze the core to the unearthly densities required to bring about the fission reaction. He also made the critical suggestion that implosion could be made much faster by increasing the ratio of the explosive in the shaped charges to the nuclear material. The faster assembly would not only prevent pre-detonation or a fizzle by making sure that the material has already reached critical mass before the nuclear fission reaction has been initiated, but the higher explosive to fissile material ratio would mean that you would need to use less of exceedingly precious enriched uranium or plutonium. In fact, as Johnny discovered because of his friend Teller who was very knowledgeable about the behavior of materials at high pressure, at the pressures that would exist in the core, materials would get squeezed so hard that they would become not just critical but supercritical, greatly accelerating the efficiency of the resulting explosion.
Because of his prestige and confidence in his calculations, von Neumann breathed fresh air into what was until then a creaky, uncertain program that Neddermeyer had been stubbornly sticking to. Fermi, Bethe and other senior scientists who had until then been skeptical of Neddermeyer’s scheme not only trusted von Neumann but regarded his dazzling mind with an awe that would be extraordinary for men who themselves were some of the leading scientific minds of the 20th century. Perhaps the ultimate tribute to von Neumann’s intelligence was paid by Bethe when he remarked after the war that “Von Neumann’s mind seemed to indicate that he belonged to a new species, an evolution beyond man.”, and Fermi once told his student Richard Garwin that von Neumann left him feeling he knew no mathematics at all. After von Neumann’s trip Charles Critchfield, a theoretical physicist on Oppenheimer’s team, said, “Johnny woke everyone up. He was a very resourceful person, at least twenty years ahead of his time.”
Once von Neumann made his suggestions, it was quite clear that the implosion program had to be given top priority. There were two main challenges associated with implementing it. One was to work out the complicated nonlinear hydrodynamical equations associated with the shock wave. The other was to find explosive materials which would sustain an imploding wave and have it impinge on the center of the fission target uniformly. To aid with the latter task, Oppenheimer invited George Kistiakowsky from Harvard who was a world-class expert in explosives; in his spare time Kistiakowsky would use his explosives knowledge to raze trees and create ski slopes on the mesa for recreation for the scientists. He also set aside separate groups that would develop the diagnostics and assembly techniques for implosion, including the development of novel detonators that would assure an instantaneous detonation on multiple points of a sphere. None of these innovations or work was trivial, but von Neumann left everyone with the feeling that it was possible.
The biggest challenge in putting implosion into practice was to design an assembly of explosives that would ensure a symmetric shock wave – an asymmetry as little as 5% would lead to a “beer can experiment”. The problem with a standard explosion is that it sets off a diverging shock wave. To ensure that this wave impinged on the uranium or plutonium at the center, it had to somehow be turned from diverging to converging. How to achieve this? At this point another valuable character showed up, from England. James Tuck was part of the British contingent to Los Alamos, a small cadre of highly accomplished physicists who were to help with the bomb. The proof-of-concept for fission and critical mass had actually been worked out in England before the United States, so it was natural for British scientists to be involved. Tuck had experience working with explosive materials of differing densities. College physics says that when a light wave passes through materials of varying densities, it bends or refracts differently. Similarly a shock wave would “refract” or bend differently with different densities. And just like a material that refracts and transmits light is a lens, so an explosive that bends and transmits shock waves is an “explosive lens”. Tuck suggested an ingenious arrangement in which a diverging shock wave could be turned into a converging one by layering explosives burning at different rates together. By starting the detonation in fast-burning explosive and then maneuvering the resulting wave inside using shaped charges of slow-burning explosives, thirty-two different waves from thirty-two different points of detonation could be made to converge to a pinpoint at the center, squeezing the material to unearthly densities and triggering the fission reaction. It was again von Neumann who worked out the mathematics of explosive lenses and played a major role in their design.
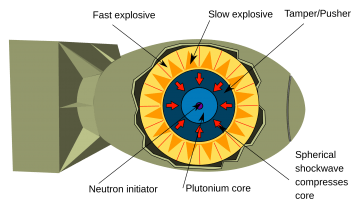
The real role played by von Neumann, Tuck, Kistiakowsky and others became apparent when a critical observation made it clear that implosion would be the only possible way to assemble the plutonium weapon. In the fall of 1944, Emilio Segrè, a protege of Fermi’s, discovered that the plutonium-239 being used in the bomb contained an isotope, plutonium-240, that was an inevitable byproduct of the reactor-bred plutonium in the reactors at Hanford, Washington state. Pu-240 underwent spontaneous fission without any initiation, and it turned out that the gun method that was being used for the uranium bomb would be too slow to prevent pre-detonation or a fizzle. Implosion would be the only possible way to go for plutonium. Without implosion the plutonium bomb would likely have been abandoned at this stage. Implosion was still considered such a novel concept that the design was tested in New Mexico’s Alamogordo desert on July 16, 1945.
Von Neumann wasn’t done with his ideas though. A crucial albeit rather dark contribution that he made was to simplify the calculations used to estimate the pressures from a bomb. Von Neumann knew that for large explosions, the effects of the detonation are not dictated by the length of the pulse but only by the excess pressure that results; this made the relationship between bomb yield and effects a much simpler one. More importantly, using his knowledge of shock wave reflections, Johnny helped to estimate the optimal height at which a nuclear bomb should be exploded to cause maximum damage. On August 6, 1945, the Little Boy uranium bomb exploded at a height of about 1900 feet on top of Hiroshima. About 80,000 to 100,000 people were instantly killed, providing a real-life demonstration of von Neumann’s calculations.
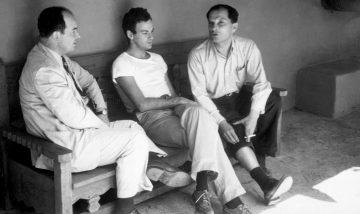
Johnny enjoyed being at Los Alamos. He reconnected with his old friend Edward Teller and invited a new friend, Stan Ulam, to help with the hydrodynamics calculations. Ulam was almost as versatile a mathematician as Johnny and the two were best friends – significant parts of Ulam’s wonderful memoir, “Adventures of a Mathematician”, are dedicated to describing conversations and travels with Johnny. They tried to recreate the famous cafe atmosphere in Eastern Europe that had fueled late night mathematical bull sessions in the early 20th century before it all came crashing down. In keeping with his predilection for wearing expensive, tailored three-piece suits, Johnny once wore one even when riding a mule in the Jemez mountains near the lab. He also went for walks with Richard Feynman, and in his own memoir “Surely You’re Joking Mr. Feynman”, Feynman points out that it was von Neumann who planted the idea in his mind that knowing how uncertain the consequences of our actions are, you should not have them weigh too heavily on your conscience. Knowing the rather grim endeavor that the physicists were involved with, this was psychologically soothing advice for Feynman.
“I am thinking about something much more important than bombs; I am thinking about computers.”
For all his critical ideas, the most important and far-reaching thing to come out of von Neumann’s work at Los Alamos had little to do with bombs. Once Johnny suggested using spherical implosion, it quickly became clear that the calculations involved would be too complex for individuals to perform. In England and before, Johnny had already been introduced to early computing machines, and he had been impressed with Alan Turing’s seminal paper on Turing machines and even tried to recruit Turing as his assistant when Turing visited Princeton to finish his PhD before the war. Now when it became clear that computing machines might be needed to handle the complex physics of implosion, Johnny was again in the right place at the right time. Stan Ulam who came to Los Alamos on Johnny’s invitation captured the problem well: “The hydrodynamical problem was simply stated but very difficult to calculate, not just in its details but even in order of magnitude.” He remembered a discussion in which von Neumann and others had suggested all kinds of ingenious shortcuts and theoretical simplifications, and he wondered whether “more simpleminded brute force, that is, more realistic, numerical work” might not be better.
To do this kind of work, the first IBM computing machines arrived in April, 1944 to help with the calculations. The implosion problem involved the integration of a hyperbolic partial differential equation in one space and one time coordinate. The integration was carried out using punched cards; Richard Feynman was in charge of this effort. Johnny himself spent two weeks programming the machines and getting a feel for the calculations. He found rewiring the tabulators especially cumbersome, and this frustration had an impact on his later crucial thinking about general-purpose computers. Johnny also knew that Howard Aiken at Harvard had had IBM build one of the first programmable computers, and he arranged for a numerical integration of a second-order partial differential equation to be run simultaneously on the Los Alamos computer and the Harvard computer as a contest; the Los Alamos computer finished first, but the Harvard computer calculated to more decimal places.
His experience at Los Alamos immediately suggested to the thinking-ten-steps-ahead Johnny that computers were going to become an invaluable asset in doing complex math and science. Quite fortuitously, around the same time he also came in contact with the pioneering engineers Presper Eckert and John Mauchly who were building one of the first pioneering general-purpose computers, the ENIAC, at the University of Pennsylvania. I have described Johnny’s accidental introduction to computers and his subsequent work as one of the fathers of modern computing in a separate essay, but it is clear that it was his work at Los Alamos that crystallized in his mind the value of computers as an enabling tool for science and technology. It was characteristically far-sighted of him that even before the war ended he was writing to a colleague, “I am thinking about something much more important than bombs; I am thinking about computers.”
Johnny’s legacy
Johnny von Neumann’s work at Los Alamos had a direct impact on the weapons of mass destruction that leveled Hiroshima and Nagasaki and that still decorate the world’s nuclear arsenals. Implosion is still the predominant technique used in fission bombs, and even in thermonuclear weapons it’s used as the primary fission device that ignites the fusion secondary. After the war Johnny kept on advising the United States government as a high-level consultant; at one point he advised every agency except the Coast Guard. He made valuable contributions not just to fission weapons but to the thermonuclear weapons that are today capable of killing hundreds of millions in a matter of minutes. Just before his death, he became one of the prime movers behind the recommendation to accelerate the United States’s nuclear ICBM program, a development that immediately made the entire world vulnerable to the threat of nuclear armageddon. He was convinced that the Soviet Union posed an existential danger to the security of the United States and advocated not just a preemptive but a preventive strike on that country; thankfully that part of his advice was not taken.
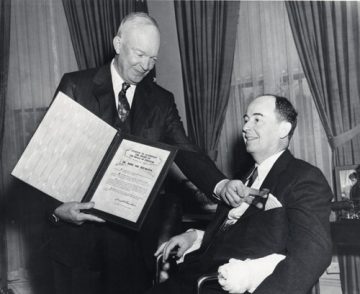
He remained a steadfast patriot, often politically at odds with his more liberal colleagues but always friends with everyone, and was held in such esteem that during the last year of his life when cancer unexpectedly struck him, he received the Medal of Freedom from President Eisenhower and spent his last days in a special, guarded suite at Walter Reed Hospital that Eisenhower had specially requisitioned for him. On his deathbed lay a manuscript of a set of lectures comparing the computer with the brain. In it lay ideas whose seeds germinated at Los Alamos and whose branches now extend into artificial intelligence, robotics and neuroscience. Perhaps his most enduring idea in this regard, and one which is very much still waiting to play out, was the idea of self-reproducing automata which could be sent out into space and would populate the cosmos with a diversity of exploding life.
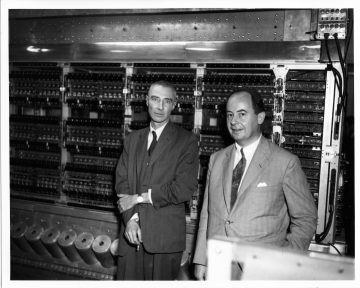
As the eminent historian of science George Dyson put it in his superb book “Turing’s Cathedral”, “Bombs made computers, and computers made bombs.” If designing the implosion lens for nuclear weapons were to be Johnny’s biggest legacy from Los Alamos, it would be a morally dubious one. But his unexpected recognition of the value of computers takes his contributions to a completely new level. While initially he did encourage using computers to simulate the workings of first fission and then fusion weapons, he made seminal contributions to charting out the stored-program concept, random access memory and what is today called the von Neumann architecture. With a talented team of engineers he designed a pioneering computer at the Institute for Advanced Study. Using this computer, his team made forays into a remarkable number of important and fascinating topics: weather simulation, artificial life, fundamental mathematical research, geophysics. The branches that these explorations sent out continue to thrive.
Johnny von Neumann’s Los Alamos story shows us that often, the most important impact of a new technology is another new technology. Often this new technology is wholly unanticipated. When Johnny came to Los Alamos, he and his colleagues thought they would be designing bombs, but what they didn’t know was that they would need computers to design those bombs. And that the computers would be far more important than the bombs even in the short term. Ultimately a hundred or a thousand years from now, if humanity still survives and the world’s nuclear arsenals are a dim memory in a history textbook, we will still be using computers, and computers will still be using us.
Recommended further reading:
- John von Neumann and the Origins of Modern Computing – William Aspray
- Critical Assembly: A Technical History of Los Alamos during the Oppenheimer Years, 1943-1945 – Lillian Hoddeson, Paul Henriksen, Roger Meade and Catherine Westfall
- John von Neumann: Selected Letters – Edited by Miklós Rédei
- The Making of the Atomic Bomb – Richard Rhodes
- Adventures of a Mathematician – S. M. Ulam
- John von Neumann – Norman Macrae
- Prisoner’s Dilemma – William Poundstone
- What John von Neumann really did for modern computing – Ashutosh Jogalekar
- Von Neumann in 1955 and 2020: Musings of a cheerful pessimist – Ashutosh Jogalekar
- The Unparalleled Genius of John von Neumann – Jørgen Veisdal
- John von Neumann – 1966 documentary by the Mathematical Association of America that includes great recollections by Johnny’s eminent colleagues (Eugene Wigner, Hans Bethe, Paul Halmos, Herman Goldstine, Edward Teller and others)