by David Kordahl
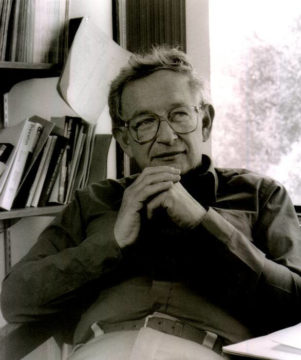
The physicist Philip W. Anderson, winner of the Nobel Prize in 1977, has lingered in the broader scientific imagination for two main reasons—reasons, depending on your vantage, that cast him either as a hero, or as a villain.
The heroic Anderson is the author of “More Is Different,” the 1972 essay that wittily dismisses the idea that the laws of physics governing the microscopic constituents of matter are by themselves enough to capture the full richness of the world. His vision of science as a “seamless web” of interconnections led to his becoming one of the public faces of so-called “complexity science,” and a founding member of the Santa Fe Institute.
The villainous Anderson is remembered for taking this position—the position that the low-level laws of physics do not exhaust fundamental physics—in front of Congress. Anderson’s tart exchanges with Steven Weinberg before the Senate debating the merits of the Superconducting Super Collider (SSC) begin a new biography, A Mind Over Matter: Philip Anderson and the Physics of the Very Many, by Andrew Zangwill. When the SSC was canceled, Anderson, who argued that the funds would be better spent on a wider variety of projects, became a target of physicists’ ire, despite his lack of any significant political influence. (Weinberg’s last book of essays, which I reviewed, extensively discussed the politics of the SSC.)
But Anderson, who died just last year, was much more than just a hero or villain. A Mind Over Matter makes the case that Anderson was “one of the of the most accomplished and influential physicists of the twentieth century.” In presenting the evidence, Zangwill, who is himself a notable physicist, gives us a tour of condensed-matter physics, the science that deals with the properties of materials not atom-by-atom but roughly 1023 particles at a time, a subject where Anderson’s influence continues on.
Since he lived so long (1923-2020), it’s inevitable that Anderson’s story covers a lot of ground. Philip Anderson was a precocious student, the son of a plant pathologist at the University of Illinois Urbana-Champaign, who left home for Harvard at sixteen. He graduated three years later with a degree in Electronic Physics, spent World War II at the Naval Research Laboratory in Washington, D.C., and returned to Harvard for his doctorate. Remarkably, he never encountered quantum theory as an undergraduate.
This period in Anderson’s development is only remarkable in retrospect. For instance, Anderson and the physicist-turned-philosopher Thomas Kuhn—who I have defended elsewhere—were competitors both as physics undergraduates and as physics graduate students sharing a thesis adviser. By the end of graduate school, Anderson was married and had a young daughter. He needed work, but the only academic position he was offered (teaching job, liberal arts) seemed unattractive. So what were his options?
Happily, he found a place at Bell Labs. Contemporary scientists might read this part of the story with envy. Anderson spent many productive years pursuing “pure science” goals at Bell Labs, without any immediate applications. His employer, in turn, benefited from such pure science, since the science of magnetic materials—this became Anderson’s early beat—was obviously relevant to engineering magnetic storage devices.
As a staff scientist at Bell Labs, Anderson was initially assigned to the lab of William Shockley, who would be remembered as one of the fathers of the transistor and, later, as an outspoken eugenicist and terrible boss. Soon, however, Anderson took on mentors whose names (Wannier, Kittel) all students of condensed-matter physics—but few others—will recognize. Zangwill gives solid accounts of the physics that Anderson explored in those years, from his studies in antiferromagnetism, where the magnetic domains within a material are ordered without exhibiting any macroscopic magnetic field, to the discovery of what came to be called “Anderson localization,” whereby disorder in a material causes electrons, described quantum-mechanically, to become trapped due to interference.
#PhysicsFactlet (200)
Anderson localization: In a periodic potential all eigenmodes will be extended. But if disorder is introduced the eigenmodes are first deformed and then become exponentially confined in a small region of the sample. pic.twitter.com/guTwpMzUGs— Jacopo Bertolotti (@j_bertolotti) February 10, 2020
In those years, Anderson developed a particular style of doing physics that sometimes put him at odds with his colleagues. In the second half of the twentieth century, many theorists enthusiastically embraced the possibilities of more powerful computers. Yet Anderson harbored a certain hostility toward this style of physics. Instead, he would often start by carefully examining whatever experimental results he could find, at times using a Xerox to magnify plots so as to better appreciate their unexpected trends. Then he would construct a simplified model to capture these trends.
Because the models had been designed to capture the dynamics of real systems, Anderson knew that his models would work for the specific experimental results he had started with. The adventure of demonstrating a model’s further merit, then, would fall to showing how well it described more phenomena than the specific data it was designed to describe. The willingness to be surprised by data was exactly the process that Anderson celebrated in “More Is Different,” which, as Zangwill points out, celebrated the possibility of “emergence” without ever using that term.
Despite working for Bell Labs, Anderson was able to travel quite extensively, which was a mark of his accomplishments, not a norm of the organization. He spent time on a Fulbright in Tokyo, and eventually became a half-time professor at Cambridge University. While at Cambridge, he taught a course on condensed matter theory that grew into his influential text, Concepts in Solids. One of the students who took this class, Brian Josephson, was inspired by the material in the course to work on the problem of two superconducting materials sandwiching a non-superconducting material, a setup now known as a “Josephson Junction,” which has been used extensively in experimental low-temperature physics, and for which Josephson was awarded the Nobel prize in 1973.
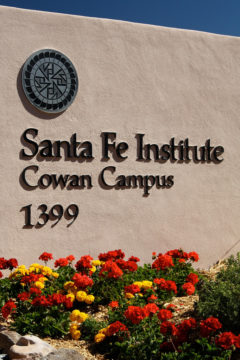
Of course, Anderson eventually won his own Nobel four years later in 1977, an award he shared with his graduate thesis adviser from Harvard, John H. Van Vleck, and an old colleague from Cambridge, Nevill Mott. (By 1977, Anderson had left Cambridge, and bounced between Princeton and Bell Labs.) But at this level of eminence, the plot becomes a bit hard to follow—which is no fault of Zangill’s, but a function of Anderson’s proliferating affiliations, with many organizations eager to claim a piece of him.
Throughout the complicated plot, Zangwill is astute in his descriptions of the way that conceptual interests, technological promise, and social pressures all compete as factors determining which problems scientists decide are worthy of their study. Problems involving superconductivity, the phenomena whereby electrical currents can flow without any resistance below a (typically very low) critical temperature, combined all these factors, and much of Anderson’s career was spent elaborating the conceptual puzzles that superconductors introduced.
One of Anderson’s early triumphs in this area involved the observation that inside a superconductor, massless particles can appear massive—i.e., photons can appear as though they have a mass. It was Anderson’s elaboration of how this could occur contextually, within the superconductor, that led Peter Higgs and others to propose a similar mechanism allowing the force carriers of the electroweak theory of the Standard Model to acquire a mass. (Anderson wrote his own account of this history back in 2015, a few years after the detection of the Higgs boson at CERN brought such issues back into the news.)
In 1986, researchers at IBM announced the existence of high-temperature superconductors, materials that became superconducting when cooled below the temperature of liquid nitrogen. Older known superconductors required much lower temperatures to reach the superconducting state. Aluminum, for instance, becomes superconducting at 1.2 K, while some high-temperature superconductors could remain superconducting at temperatures well above 100 K. Naturally, Anderson was excited.
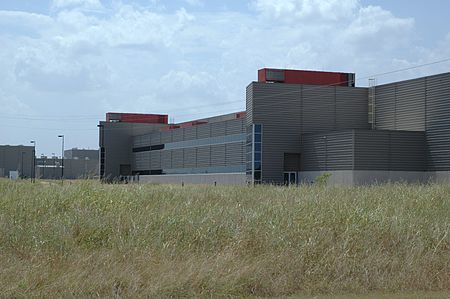
It was in the wake of these discoveries that he testified against the SSC, noting that “perhaps the first billion dollars of the $5 billion budget of the SSC might profitably be spent on materials research.” Whatever one’s position on the ultimate cancellation of the SSC, one has to admit some sense to this—the Superconducting Super Collider, after all, was certain to rely on superconductors, and in a time when their prospects seemed to be rapidly expanding, one can see why Anderson would be a partisan for his subject.
While Zangwill is generally sympathetic to Anderson, A Mind Over Matter does not hesitate to point out his shortcomings. Zangwill is especially critical of Anderson’s behavior in his late career, when his imperiousness caused him to ignore signs that he was on the wrong path regarding the high-Tc superconductors. Without ever quite saying so explicitly, Zangwill implies that Anderson’s attitudes toward his peers may have caused lasting damage to the study of superconductors, as some of his good ideas became harder for colleagues to take seriously when Anderson seemed to take no one seriously but himself.
Regardless, it’s a good thing that Andrew Zangwill has written this book. I expect that it will find many readers among professional physicists, and I hope that some students find it. In addition to being a lucid biography of one of the great twentieth-century scientists, it’s also a skeleton-key for readers interested in the physics of complex systems. We can only hope that it might fall into the hands of the next Philip Anderson.