by David Greer
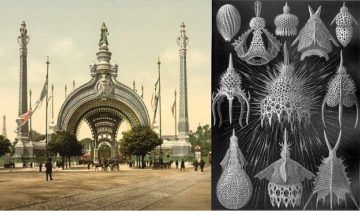
The 1900 Paris Exposition was a grand event, a world’s fair intended to honor the great achievements of the century just completed while heralding those of the century about to begin. In this spirit, architect René Binet designed a daring, futuristic entrance gate unlike anything ever seen before. It was as if he had had a prophetic vision, sixty years into the future, of a rocket launching into space. Binet’s concept, bizarre as it seemed, was far from imaginary. It was modelled after one of the most common creatures on Earth, though not one ever seen by anyone without a microscope. The animal that inspired the design of Binet’s Porte
Monumentale was a radiolarian, a form of zooplankton found in oceans everywhere.
The image of the radiolarian that so entranced Binet had been drawn by the German scientist Ernst Haeckel, a contemporary and admirer of Charles Darwin. Fascinated by the diversity of species and especially of the minute sea creatures seen through his microscope, Haeckel obsessively drew their forms in painstakingly detailed images. Reproduced in book form as Kunstformen der Natur (Art Forms in Nature), Haeckel’s images caught the attention of architects and designers and became an inspiration for the Art Nouveau movement, of which Binet’s radiolarian gate was an early example.
The radiolarians drawn by Ernst Haeckel display elaborate curves, masses of protruding spikes, antenna-like protuberances and interior chambers. The reason a microscope is required is that just about any one of those creatures, notwithstanding their complicated structures, could be contained inside the thickness of a sheet of paper or the width of a human hair—about a tenth of a millimeter. Tiny and virtually invisible as most plankton are, they make up more than 90% of the biomass in the world’s oceans and are collectively the lowest rung on the marine food chain. Everything we eat that comes from the ocean ultimately depends on plankton for its existence. As if that’s not reason enough to pay attention to the future of plankton, it’s worth considering that about half of the oxygen we take in with every breath also owes its existence to plankton.
The Life and Tides of Marine Plankton
The Greek word planktos, meaning drifter, sums up the life story of plankton. Unlike fish or marine mammals, plankton are unable to propel themselves forward or in any way resist the flow of the tide or current that carries them. Where the current moves, there they go. An airborne counterpart such as a thistle seed carried by a breeze would by definition be a form of plankton too, though the term is generally understood to refer to aquatic life—specifically, organisms that inhabit the water column above the seabed (or lake bed or river bed). Sea creatures that can fully control the direction they move (such as fish and seals) are collectively known as nekton (Greek for swimmers and a useful Scrabble word to know).
Because plankton are defined by their mobility rather than by appearance, they include a wide variety of life forms, ranging in size from a fraction of a micron (one-thousandth of a millimeter) to jellies up to two meters across. For the most part, plankton are either phytoplankton (plantlike organisms) or zooplankton (animals). Smallest of all are the bacterioplankton (bacteria) and virioplankton (viruses).
Though found throughout the world’s oceans, plankton are most abundant in nutrient-rich regions such as shallow seas, continental shelves and upwelling regions where deep ocean water rises to the surface. Plankton naturally gravitate to sunlit waters because, like plants on land, phytoplankton grow through photosynthesis, using carbon dioxide and sunlight energy to produce carbohydrates (which form the phytoplankton’s cells), with oxygen as a by-product. And some by-product it is. Infinitesimal as they are, phytoplankton produce more oxygen than all the world’s rainforests combined and roughly half of the oxygen on the planet—in other words, roughly half of the air we humans breathe.
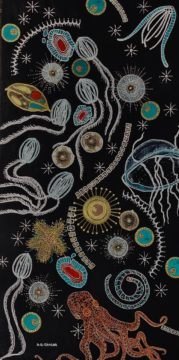
Plankton are small but so numerous (a spoonful of ocean water might be home to several thousand phytoplankton) that they are estimated to comprise more than 90% of the marine biomass. Most impressively tiny are the phytoplankton, typically ranging in size from 0.002 mm to 1 mm and comprising at least 100,000 species including cyanobacteria (sometimes called blue-green algae), algae like the diatoms that surround themselves with glass-like structures, and coccolithopores with calcium carbonate armor. The smallest and most abundant phytoplankton species, Prochlorococcus, was only discovered in the 1980s. At the very bottom rung in the food chain, phytoplankton are preyed upon by zooplankton (animal rather than plant forms of plankton), which in turn become food for fish, crustaceans and larger animals. If phytoplankton migrate or are displaced from a region, the creatures that depend on them for food must inevitably follow if they are to survive.
Quantity is important when you’re the lowest rung in a food chain with predators with appetites as prodigious as the blue whale, whose daily diet may include up to four tons of krill, a small shrimplike crustacean generally included in the definition of plankton. The blue whale (like other baleen whales such as the humpback) wastes no time restoring nutrients to the marine food cycle through defecation that nourishes other plankton populations. The rebounding of whale populations following international whaling bans has done much to restore an ocean ecosystem cycle that depends as much on its largest species as on its smallest.
Once a phytoplankton, always a phytoplankton. An alga remains an alga until it is eaten or otherwise encounters its natural demise. Not so with all zooplankton. Zooplankton such as copepods live out their brief, predatory lives as plankton, but zooplankton also include a wide range of eggs and larvae that eventually mature into creatures such as crabs and octopi and sea stars that, once they have attained mobility, will cease to be ocean drifters and relocate to new locations such as the seabed, in the process transforming from plankton into nekton (swimmers rather than drifters). Zooplankton are also generally considered to include krill, shrimplike crustaceans that populate the seas in vast numbers, as well as jellies.
From Retractable Oars to Byzantine Domes—The Bizarre Improbability of Plankton Shapes
Most species evolve a shape that best enables them to survive and prosper in their habitat. Fish gotta swim and birds gotta fly, so fish are typically streamlined and elongated, the better to move quickly through water, while birds have evolved a frame that minimizes wind resistance. Evolution rewards shapes that promote survival, whether for capturing prey or avoiding predators.
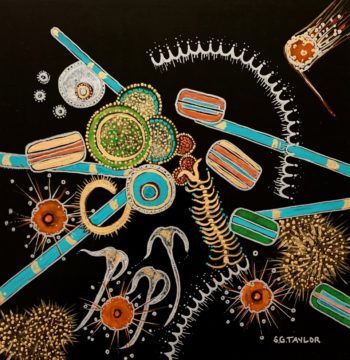
The shapes of many plankton suggest no purpose at all. They’re as varied as boomerangs and yoyos and Byzantine towers. As drifters in the tides, plankton have no need to be streamlined or to appeal to potential mates or in any way appear either pretty or functional for any other purpose than photosynthesis. But while they’re unable to adjust their horizontal position, plankton can migrate up and down in a vertical column of water, a few millimeters at a time yet sometimes hundreds of meters a day, from darkness to light and back again, for nutrition or security depending on the time of day. Some plankton have evolved retractable paddles and oars that enable them to climb through water. Others, like jellies, have built-in buoyancy mechanisms to keep them afloat. The spines and domes on the radiolarians sketched by Haeckel likely create drag that discourages sinking and may have the added benefit of making predators think twice about consuming them.
Everything in nature theoretically evolves for a purpose, but the bizarre protuberances on many plankton give one cause to wonder. It’s almost as if some features evolved as a trial run, served no obvious useful evolutionary purpose, but never disappeared because they had no negative effect on the life of an ocean drifter. The net result is that plankton appear in bizarre shapes sufficiently varied and numerous to have kept an army of Ernst Haeckels busy drawing them through several lifetimes.
If Not for Plankton…
In addition to being the anchor for the marine food chain and a primary source of the world’s oxygen, plankton is the little-known author of many other natural phenomena of greater or lesser importance:
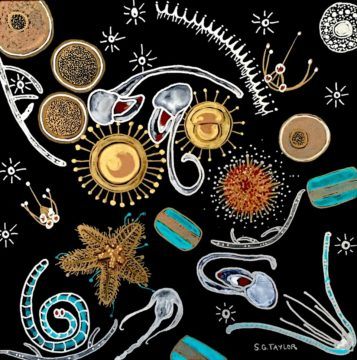
· The tangy scent of sea air. The characteristic smell of the ocean that draws you to the beach is that of dimethyl sulfide (DMS), a gas released by the decomposition of algae by bacteria. DMS released into the atmosphere resulting from the breakdown of ocean algae is also the source of particles that contribute to the formation of clouds.
· The colour of the ocean. Plankton can be found in saltwater and freshwater. One way to tell if a body of water has a large plankton population is to look at its clarity. Very clear water usually has less plankton than water that is more green or brown in color. As Rachel Carson observed in The Sea Around Us, “The deep blue water of the open sea far from land is the color of emptiness and barrenness; the green water of the coastal areas, with all its varying hues, is the color of life…. Where the water is rich in plankton, it loses the glassy transparency that permits this deep penetration of the light rays. The yellow and brown and green hues of the coastal waters are derived from the minute algae and other microorganisms so abundant there.”
· Sea foam. White sea foam that piles up on beaches after a storm is usually a product of decaying algal blooms offshore.
· The white cliffs of Dover. The massive calcite cliffs on the south coast of England are composed almost entirely of the remains of single-celled coccolithophores deposited on what was then a seabed millions of years ago.
· Red tide. Red tides and other toxic algal blooms result from a massive increase in numbers of certain types of phytoplankton that release dangerous toxins.
· Bioluminescence. Many planktonic creatures produce short flashes of light when disturbed or attacked. The resulting bioluminescence can often be seen in breaking waves and can be so spectacular that the whole surface of the sea appears to sparkle at night.
· Oil and gas deposits. The dead remains of plankton sinking to the seabed over millions of years led to the sequestration of carbon dioxide in sedimentary rocks as organic carbon, some of which becomes oil and gas when heated and compressed.
Interesting as these facts may be, what really matters most about plankton, at least insofar as their importance to humans is concerned, is the fact that they enable us to breathe. If anything were to wipe out the vast stores of plankton in the oceans, that would be the end of the human race as well as any other species that depends on the availability of oxygen for its survival.
Perils Facing Plankton
In 2015, Margaret Atwood summarized the direst consequences the world is likely to encounter if the struggle to wean ourselves off fossil fuels does not go well. Among them she included this observation: “Most lethal to us — and affected by warming, acidification, toxins, and dying marine ecosystems — would be the destruction of the blue-green marine algae that created our present oxygen-rich atmosphere 2.45 billion years ago, and that continue to make the majority of the oxygen we breathe. If the algae die, that would put an end to us, as we would gasp to death like fish out of water.”
Before the impact of greenhouse gas emissions became such a dominant issue, human impacts on the ocean of greatest concern had to do with overfishing and pollution. One notable form of pollution in terms of its impacts on plankton life is the decades-long spread of plastic waste throughout the oceans. Over time, plastic drifting in the oceans breaks down into microscopic particles that become attached to plankton, thence entering and contaminating the entire range of species, including humans, that are part of the marine food chain. In addition, plastic waste interferes with plankton photosynthesis by blocking phytoplankton access to sunlight.
Climate change inevitably impacts species at all levels, and plankton are no exception. A 2019 study (Jonkers et al) found a correlation between ocean warming and the gradual and continual migration of zooplankton communities towards the cooler waters of polar regions. Large-scale plankton migrations inevitably lead to migrations of fish that feed on zooplankton, which may be why fish considered to be warm-water species, such as bluefin tuna and anchovies, are now commonly found in waters of the North Atlantic. (The theory of large-scale plankton migration has more recently been challenged by Trubovitz et al [2020], who argue that some plankton species are unable to migrate in response to changing temperatures and instead risk becoming extinct.) In addition to geographical changes, the seasonal timing of the occurrence of plankton is also changing as the seas warm, significantly affecting species such as cod, the survival of whose larvae depends on the availability of certain species of plankton at certain times. Cael et al (2021) conclude that climate-driven shifts this century, being often abrupt, large in amplitude and extent, and unpredictable using standard early warning signals, will particularly affect phytoplankton, especially fast-growing species such as diatoms.
While changes in the location of plankton and the timing of their life cycles may have a direct impact on fisheries regimes, of even greater potential consequence is another phenomenon associated with global warming—the effect on ocean acidity of increasing atmospheric concentrations of carbon dioxide. Ocean acidification, sometimes referred to as global warming’s evil twin, played a role in at least two of the five major mass extinctions in the history of life on Earth and is already beginning to have a significant impact on biodiversity in the oceans.
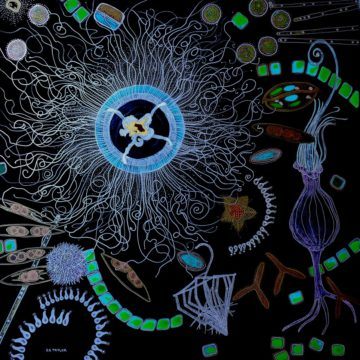
The oceans absorb about 30 percent of carbon dioxide released into the atmosphere. The more carbon dioxide the ocean absorbs, the greater the acidity at the surface of the ocean. On the pH scale, which runs from 0 to 14, 7 is neutral, anything higher than 7 is considered alkaline, and anything lower than 7 is considered acidic. The ocean’s average pH is currently 8.1 and gradually dropping as the amount of absorbed carbon dioxide increases. That places at risk any creature that produces forms of calcium carbonate to create its shell or skeleton, such as oysters and corals and pteropod molluscs, a type of zooplankton that is a major component of the Antarctic food web. The U.S. National Oceanic and Atmospheric Administration had this to say about the impact of acidification on pteropods: “When pteropod shells were placed in sea water with pH and carbonate levels projected for the year 2100, the shells slowly dissolved after 45 days.” The implications for a broad variety of ocean creatures and on those that depend on them for survival are unsettling.
But the species of greatest concern may ultimately be the least visible and most primitive—phytoplankton such as single-cell algae on which all marine life depends for its food and on which all land animals depend for the breath of life. As the ocean absorbs more carbon dioxide and its pH edges closer to the acidic end of the scale, the ability of phytoplankton to convert carbon dioxide through photosynthesis will become ever more critical. In 2021, global energy-related carbon dioxide emissions rose by 6% in 2021 to 36.3 billion tonnes, their highest ever level. In 2010, a team of researchers from Dalhousie University calculated that global phytoplankton numbers were dropping by about 1% per year (Boyce et al, 2010). The warmer the ocean becomes, the greater the likelihood of further reduction in phytoplankton numbers, thanks in part to a reduction in nutrient supplies from the deep ocean as rising temperatures affect the flow of currents and stability of the upper ocean.
This is not to suggest that you ruin your day by adding plankton troubles and the availability of oxygen to your list of doomsday concerns. After all, you can’t go out and plant a billion plankton the way you might a million trees to absorb some of that pernicious carbon dioxide. But whatever you may choose to do to help mitigate the flow of CO2 emissions to the atmosphere or to encourage the powers that be to do so, it pays to be as well informed as possible about the causes and means of mitigating the impacts of global climate change, including the very significant role of plankton.
***
Many thanks to Susan Taylor of Blood Star Gallery, South Pender Island, Canada, for graciously permitting the use in this article of paintings from her Importance of Plankton series.
References
Atwood, Margaret. It’s not climate change—it’s everything change. Matter, 27 July 2015.
Bagusche, Frauke. The Blue Wonder: Why the Sea Glows, Fish Sing, and Other Astonishing Insights from the Ocean. Vancouver: Greystone Books, 2021.
Boyce, D., Lewis, M. & Worm, B. Global phytoplankton decline over the past century. Nature 466, 591–596 (2010).
Cael, B. B., Stephanie Dutkiewitz and Stephanie Henson. Abrupt shifts in 21st-century plankton communities.Science Advances. 29 Oct 2021 Vol 7, Issue 44
Carson, Rachel. The Sea Around Us. Oxford: Oxford University Press, 1951.
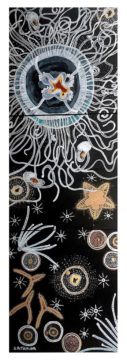
ETH Zurich. Mapping the global distribution of phytoplankton. ScienceDaily, 22 May 2019.
Haeckel, Ernst. Art Forms in Nature. New York: Dover Publications, 1974.
Jonkers, L., H. Hillebrand and M. Kucera. Global change drives modern plankton communities away from the pre-industrial state, Nature, https://www.nature.com/articles/s41586-019-1230-3 (2019)
Kirby, Richard R. Ocean Drifters: A Secret World Beneath the Waves. Richmond Hill, Ontario: Firefly Books, 2010.
Kolbert, Elizabeth. The Sixth Extinction: An Unnatural History. New York: Henry Holt and Company, 2014.
Lin, Vivian S. Research highlights: impacts of microplastics on plankton. Environmental Science: Processes & Impacts. 2016, 18, 160-163.
McQuatters-Gollop, Abigail. Jellyfish alert: increased sightings signal dramatic changes in ocean food web due to climate change. The Conversation, 24 August 2022
Morelle, Rebecca. Ocean’s hidden world of plankton revealed in ‘enormous database’. BBC News, 22 May 2015.
National Oceanic and Atmospheric Administration, U.S. Department of Commerce. Ocean acidification. N.d.
Savoca, M.S., Czapanskiy, M.F., Kahane-Rapport, S.R. et al. Baleen whale prey consumption based on high-resolution foraging measurements. Nature 599, 85–90 (2021). https://doi.org/10.1038/s41586-021-03991-5
Trubovitz, S., Lazarus, D., Renaudie, J. et al. Marine plankton show threshold extinction response to Neogene climate change. Nat Commun 11, 5069 (2020). https://doi.org/10.1038/s41467-020-18879-7