by Paul Braterman
This book is a delight. Drawing on a deep love of her subject matter, the author interweaves descriptions of a sequence of rock types with the story of her own personal and professional life. An extremely difficult feat to bring off, but Marcia Bjornerud (M) manages to do this without artificiality or mawkishness, drawing on historical and philosophical insights whose origins are hinted at throughout the text, and explaining complex concepts in lucid and enjoyable language. The only thing I did not like about the book is its appearance, which for me fails to suggest the hard-won insights that it is so successful in conveying.
M began her training in the early 1980s, at an interesting time, when the community of geologists was still absorbing the implications of plate tectonics, and geology was changing from a descriptive to an explanatory science. This was also when, despite the best efforts of the fossil fuel companies, predictions of global warming were beginning to seep into public awareness. A difficult situation for a socially aware geologist, since these same companies are going to be the main employers of her students.
She was established in what most people would regard as a successful academic career, having earned tenure at an unnamed but easily identified major Midwestern research university. But she was not happy, either with the location (in the middle of a vast expanse of one of the few kinds of rock that she really seems to find boring) or with the atmosphere of the place. And so, despite having got away with such subversive activities as smuggling a mention of Gaia into a lab manual chapter on biogeochemistry, and devising undergraduate classes that actually taught students something, she decided that she needed to move. Easier said than done. She was too far along in her career for ordinary entry-level positions, but not yet advanced enough for senior appointments. So she made the extraordinary decision to apply for a teaching job at Lawrence University, a tiny private liberal arts college in Wisconsin, and we must be glad that she did so.
Lawrence is clearly a very special kind of place. It educates not only its students (all 1500 of them), but its faculty, by involving all of them in its “great books” programme. And there, released from the treadmill of writing papers to gain grants to fund students to collect data to write papers, she has been able to explore at will and meditate on her findings, in the occasional specialist publication but also other outlets such as newspaper articles, and in a series of books including Timefulness, which I reviewed here earlier, and the present volume. (All this despite an unusually demanding family situation, and the responsibilities of rebuilding her department.)
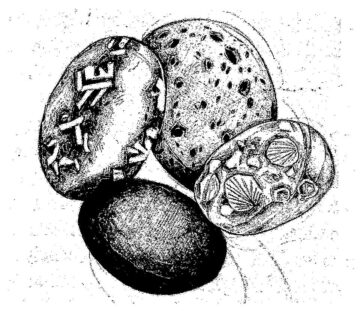
I will not attempt to summarise M’s reflective personal narrative, and can only give the sketchiest outline of the book’s wide-ranging scientific content. We start with the sandstone of her Wisconsin childhood, the highly processed final erosion product of Proterozoic mountains. The rock has a highly porous texture, which does not retain nutrients, making the soil a poor basis for long-term agriculture once the woodlands had been so enthusiastically cleared. Its uniformly spherical impervious grains are now in great demand for holding cracks open in fracking, and farmers are finding that selling their subsoil is more lucrative than cultivating it. Basalt, which comes next, is just magma, upwelling on earth from beneath the crust, liquefying1 as it rises to the surface, and then solidifying as it cools, but on the moon exactly the same kind of stuff, cooled down shortly after its formation. The most unprocessed of rocks, pouring out of volcanoes, or being generated where plates move apart at mid ocean ridges. But even so there are interesting variations, depending on local changes in composition and on how fast the material cooled down, dictating its texture, and the presence or absence of separate distinct crystals. Tuff is a very different kind of volcanic rock, explosively emitted at volcanoes such as St Helens, where tectonic forces push water-rich material from the crust back down into the mantle by the convergence of plates in subduction zones. (I know that I haven’t explained the concepts of tectonics, crust, mantle, plates, convergence, and subduction, or why water makes such a difference. M does.)
Next comes diamictite (not perhaps an everyday word), a jumble of fine-grained rock and ill-sorted pebbles, the solidification product of the mud and rubble swept along by glaciers. This has a special meaning for M, because it is the rock that she studied on Spitsbergen for her PhD. The rock there showed a rather surprising feature, a sorting out of layers into coarser and finer material, indicating seasonal variation. So this material was not deposited on land, but carried there in icebergs calved each summer from the glaciers. Moreover, the flatter pebbles tended to point more in one particular direction, showing the effect, long after the rock had solidified, of being pushed that way by mountain-building processes. It was the sorting out of this complex story that started M on her university career.
Among the many kinds of rock at Spitsbergen are turbidites. These are thick layers of sediment laid down one after another when underwater avalanches at the edge of continental shelves sweep material down into the abyss, and have a distinctive structure corresponding to the crude sorting that takes place as the material settles. When continental plates come together, this material is forced up again, and like other rocks can then be further deformed and tilted by tectonic movements.
One particularly famous exposure of turbidites is at Siccar Point, where such rocks have been tilted, eroded, and millions of years later covered by a layer of sandstone. I was glad to see M placing the site in its proper context, not as the place where Hutton discovered deep time, but as a striking confirmation of the theory that he had already adopted, while meditating on the earth as he went about his business as a farmer. Here I would add that we have in Hutton’s 1785 introduction to his work (mercifully brief, compared with his full, 1788, Theory of the Earth) a most interesting statement of his motivation. As a deist, he believed that the earth had been created for long-term human habitation. But he could observe erosion of the soil, so there must be some processes at work to replenish it. And so we have him searching in the rocks for confirmation of his thesis of recycling, to see how the same kinds of process that are still at work today contributed to regeneration in the remote past. As M points out, not in accord with the middle school “scientific method,” and I would be even more emphatic than she is in rejecting that dreary doctrine. We must allow a research programme to build on its successes, before we challenge its shortcomings.
M discusses two specific philosophical concepts of importance to geologists. Firstly, uniformitarianism, Hutton’s working assumption that the present is key to the past. This was formalised by Charles Lyell, whose Principles of Geology was subtitled “An attempt to explain the former changes of the earth’s surface by reference to causes now in operation.” This need not imply that the scale or intensity of causes has always been the same as they are now. Even so, M warns against this principle becoming a mental straitjacket, pointing to changes in continent-building style during Earth’s first billion years, and to the reluctance of some of her colleagues to accept the impact hypothesis (which I discussed here) for the demise of the dinosaurs. Secondly, T. C. Chamberlin’s “Method of Multiple Working Hypotheses, put forward in an 1897 paper which she cites. Here Chamberlin attacks the very concept of the scientific method, especially when it focuses on one hypothesis, and advocates thinking in terms of multiple hypotheses, as the title of the paper tells us, each of which will be supported or modified by different data. This, he says, is particularly applicable to geology, where the phenomena are complex and more than one cause may be involved, including causes that have not yet even been considered.2 Right now, I wish I could make Chamberlin’s essay compulsory reading for all the pundits who are telling us the reason for the outcome of the US Presidential election. And while writing this, it occurred to me that much the same could be said about the extinction of the dinosaurs. Here there was for many years a fierce conflict (in which M describes herself as agnostic) between advocates of the impact hypothesis mentioned above, and the hypothesis that it was caused by massive volcanic activity known to have occurred at around the same time, but perhaps we should not regard these as mutually exclusive alternatives.
Dolomite is the closest thing there is to a rock that M doesn’t like. Hard, white, very difficult (in my experience) to break, remarkably unreactive given its chemical composition, and stretching unfolded for hundreds of miles in every direction from the research university I mentioned earlier. Chemically very simple (it’s a calcium magnesium carbonate), the only interesting thing about it is that it is extremely difficult to prepare in the laboratory. On current thinking, its formation involved the action of sulphur-metabolising bacteria, and laboratory synthesis only takes place in the presence of bacterial slime. This biological involvement in the formation of a mineral is by no means unusual. Chalk and other limestones are formed from the shells of living things, but what has only recently been realised is that over 40% of all the different kinds of mineral identified on earth owe their origin, at some stage, to biological activity. As M puts it,
Animal, vegetable, mineral, and microbe are very untidily entangled; Gaia is a stranger and more complicated creature than we ever imagined.
Granite is much richer in silica and alumina than basalt, and its formation is peculiar to the earth (in this solar system, anyway). It is formed from the lowest melting point components of magma, after other rocks have crystallised out, although the process is still not fully understood. Examination of the oldest granites suggest that they were formed in the first billion years, before Earth developed its current system of plate tectonics. We know from dating craters that the moon, and by implication the earth, was subjected to heavy external bombardment between 4.2 and 3.8 billion years ago, and M is attracted to the idea that this bombardment may have contributed to early granite formation, through chemical processing of submarine craters.
While discussing granite, M goes out of her way to acknowledge the massive contributions of Arthur Holmes, from his very early use of radiometric dating to the development by the 1930s of a version of plate tectonics. A valuable corrective to the opinion, widespread among US geologists, that this concept only dates from the 1960s. She is however mistaken in describing his 1911 paper as the first use of radiometric dating. Here the credit must go to Boltwood (19073; discussed in context here), in collaboration with Ernest Rutherford, no less.
Eclogite is a beautiful rock, studded with garnet. It is produced in subduction zones at high pressure, but relatively low temperature, and is much denser than the basalt from which it is formed. Indeed, this density plays an important role in dragging material down, driving the process of plate tectonics, just as Holmes had suggested . M herself studied an interesting example, produced during the coming together of Pangea and the uplifting of the Appalachian-Caledonian-Scandinavian mountain ranges. A detective story, with several twists in the plot, involving the role of water in eclogite formation, deep earthquakes caused by the presence of unusually strong dry rock,4 the partial conversion of this rock to eclogite, and a rare glassy mineral5 formed by intense frictional heating in the earthquake zone. Earthquakes occur along fault lines, where blocks of crust are sliding past each other, but only some fault movements create earthquakes or glass, depending on the rigidity and brittleness of the rocks at depth. Such complexity has its own kind of beauty, very different from the much-lauded austere beauty of physics, but much more relevant to our own urgent needs as earthlings. A much more common kind of non-crystalline mineral is chert, formed from the silica-containing remains of minute marine organisms. Flint is a form of chert as it occurs in sediments, and has been used for toolmaking since the dawn of humanity.
For her last topic, M makes us join a group of students examining an exposure of quartzite, which has preserved ancient layering and ripple marks. Where the rocks have been bent, the layers have slid over each other, just as pages in a book slide over each other when curved (I have seen this, of course, countless times, but had never before thought about what I was seeing.) Quartzite is the dense pale rock that sandstone is converted to when it happens to get buried, rather than eroded. And exposed again later, in this case one and a half billion years later, while in the meantime supercontinents have been and gone and life has evolved from simple micro-organisms to what it is today.
In a brief epilogue, M contemplates the diversity of pebbles on the beach at Lake Superior. Here we see the products of communication between mantle, crust, and atmosphere. Subduction returns crustal material to the mantle, volcanism reverses the process, tectonic uplift turns ancient seabed into mountain ranges, biological activity links soil and atmosphere, deep metamorphism responds to surface rainwater, and the surface has been repeatedly reworked by Ice Age glaciers, both recent and in the remote past. Earth is a multilayer living system and it is our home (there is a brief earlier reference, now painfully relevant, to those who harbour banal fantasies of terraforming Mars). As she says, what a place to grow up.
Let me conclude this review by quoting, as M quotes, the recurring question that she retains from her Lutheran catechism, long after she has lost faith in Luther’s answers, and now more urgent than ever; what does this mean for us?
***
1] Basalt, like most materials, is denser when solid, and becomes more fluid when pressure is released. Water/ice, and silica, an important constituent of granite, are among the few substances for which the reverse is true. This is why ice floats, and why we have granite plugs in volcanoes, but not granite lava flows.
2] Reading Chamberlin’s paper along with M’s book led me to an interesting example. Chamberlin mentions three possible factors that may have contributed to the formation of the Great Lakes basin. M tells us of a fourth, which he could not have even imagined. Ancient tectonic activity led to the deposition of an unusually thick basalt deposit beneath the lakes, showing up as a gravitational anomaly of which Chamberlin could have had no inkling, and creating a depression much older than the glacial factors that he invokes.
3] “On the Ultimate Disintegration Products of the Radio-active Elements. Part II. The Disintegration Products of Uranium,” Boltwood, B. B., Amer. Jour. Sci. 23 (1907). 77 – 88. The idea that Holmes was the first to do this comes from Cherry Lewis’s adulatory biography, The Dating Game.
4] Granulite. As M explains, earthquakes are characteristic of the deformation of very strong rock. More easy-flowing rocks simply deform during tectonic processes, but the strongest store up stress energy until they fracture catastrophically.
5] Pseudotachylite