by Ashutosh Jogalekar
What’s the universe made up of? Most people who have read popular science would probably say “Mostly hydrogen, along with some helium.” Even people with a passing interest in science usually know that the sun and stars are powered by nuclear reactions involving the conversion of hydrogen to helium. The dominance of hydrogen in the universe is so important that in the 1960s, two physicists suggested that the best way to communicate with alien civilizations would be to broadcast radio waves at the frequency of hydrogen atoms. Today the discovery that the stars, galaxies and the great beyond are primarily made up of hydrogen stands as one of the most important discoveries in our quest for the origin of the universe. What a lot of people don’t know is that this critical fact was discovered by a woman who should have won a Nobel Prize for it, who went against all conventional wisdom questioning her discovery and who was often held back because of her gender and maverick nature. And yet, in spite of these drawbacks, Cecilia Payne-Gaposchkin achieved so many firsts: the first PhD thesis in astronomy at Harvard and one that is regarded as among the most important in science, the first woman to become a professor at Harvard and the first woman to chair a major department at the university.
Donovan Moore has performed a service in bringing this remarkable woman’s life story to a broad audience, and he tells it with sensitivity and a wonderful sense of place. His story focuses on the human personalities, and while I was a bit disappointed that the book is way too light on the science, it is still a worthy read. Payne grew up in a quintessentially Edwardian England in the late 19th century, a time when women were all supposed to be like those in “Little Women”, grooming themselves to be proper girls who spent all their time cultivating skills that would make them prime prospects for marriage to a wealthy man. But Cecilia was different from the start, largely because of her parents; she did not care much for looks and dresses and much more instead for exploring nature and playing with her two siblings. Her doting father, a lawyer, writer and musician who was fifty-five when he had her, spared no efforts to get her interested in science, music and books.
Sadly he died when Cecilia was four. But Cecilia’s mother Emma was no less encouraging. She was a talented copyist who came from an academically inclined family and made a living copying J. M. W. Turner’s paintings. She also spared no expense in letting Cecilia indulge her interests in everything from the classics to Latin and Greek. Shortly after her husband’s death Emma moved the children to London from the rural countryside that Cecilia loved so that they could have many more opportunities. But Emma did not know much about science, and it was only through an accidental discovery of a family member’s books on botany that Cecilia acquired her first love of science. She spent time arduously translation Carl Linnaeus’s classification system. If she had not become an astronomer she almost certainly would have become a botanist.
Throughout her childhood education Cecilia displayed the kind of rebellion and independent toughness that would characterize her life and career. She kept on asking to study more in grade school, even asking to take tests that were not recommended, until her mother was informed that the school did not have the kind of resources needed to satisfy her intellectual curiosity. Fortunately Cecilia and Emma had a stroke of luck in finding St. Paul’s Girls School in London which was run by a woman named Frances Gray, a graduate of Newnham College at Cambridge, who believed that women should try to educate themselves well enough so that they could work and support their families. At St. Paul Cecilia flourished, and among other things learnt music from Gustav Holst who was employed as a music teacher there. She did exceedingly well and was accepted at the only women’s college at Cambridge, Newnham, in 1919.
Cambridge was still a very male-dominated establishment; they wouldn’t issue degrees to women until after the Second World War. A proposal to award formal degrees to women almost led to a riot resulting from a march by several men who broke down the gate at Newnham’s entrance while its female head calmly blocked their way. But in spite of this discouraging environment, Cecilia also happened to arrive at Cambridge at the right time, one of the most revolutionary in the history of science. J. J. Thomson had discovered the electron about twenty years earlier, Ernest Rutherford had discovered the nucleus and nuclear transformations and was leading half a dozen future Nobel Prize winners at the famed Cavendish Laboratory, and Niels Bohr had just published his atomic model six years earlier. In addition Einstein had put the finishing touches to his field equations of general relativity in 1915. With the end of the war the Cambridge air crackled with a revolutionary scientific spirit and an eagerness to make up for lost time.
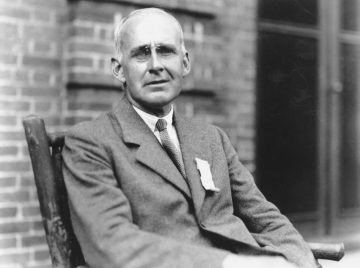
But the event that changed Cecilia’s interests from botany to physics and truly set her on her life’s course was a lecture by Arthur Eddington. Eddington was the dean of British astronomy and had just become famous for verifying one of the most important predictions of Einstein’s general theory of relativity – the bending of starlight around a star’s gravitational field. Eddington had made his measurements of the sun’s slight bending of distant starlight during an eclipse visible from the African island of Principe. Both he and Einstein had overnight become household names and partners from two countries which had just concluded a bitter war. Eddington would continue to write bestselling popular science books and turn into the foremost exponent of relativity, spreading the gospel like wildfire (He once replied when asked whether it was true that only three people understood relativity, “I am trying to think who the third person is.”) Eddington was an unusual example of a scientist who became famous not necessarily for always providing the right answers but for asking the right questions. In his long and august career as a successful scientist, two instances of his stubborn refusal to accept new theories and new evidence stand out – his rejection of Payne’s discovery that the universe is mainly made out of hydrogen and his rejection of the astrophysicist Subrahmanyan Chandrasekhar’s thesis that white dwarfs must undergo gravitational collapse beyond a certain mass limit. But these episodes are a testament to Eddington’s greatness as a scientist, since the best science inevitably involves making mistakes.
Eddington’s lecture made such a deep impression on Cecilia that she had a kind of ecstatic nervous breakdown and could not sleep for several nights. She knew she had to switch to physics but it was a risky move; because of the way the system at Cambridge was set up, if she failed her first exams in physics she would not get a second chance and would have to leave. But Cecilia did not disappoint, and along with acing the exam, she got a chance to study with Ernest Rutherford at the Cavendish and get introduced to the latest concepts in atomic physics. She did not know it, but Cecilia Payne might have been one of the very few people in the world then to acquire a thorough knowledge of both atomic physics and astronomy. It would change both her career and the course of astronomy.
It’s interesting to note a key fact about Cecilia’s career at this point, especially since as a woman she had to face many serious hurdles to advancement. While her gender did hold her back, it was thanks to farseeing men like Eddington and Rutherford that she was able to at least haltingly find her voice. Eddington and Rutherford were still products of their times – Cecilia remembers Rutherford emphasizing the “Ladies” in “Ladies and Gentlemen” as he strode into the lecture hall, accompanied by heavy stomping of feet from the preponderance of men there that made her feel like sinking into the floor – but they were much more progressive than many of their contemporaries and realized that they had amongst them a rare talent who should be encouraged as much as possible.
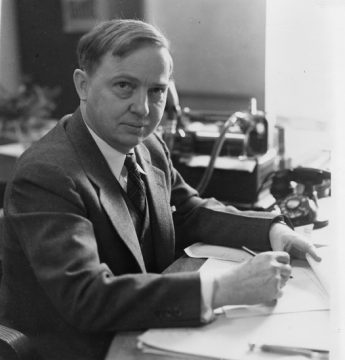
Eddington in particular encouraged Cecilia to apply to the United States. He recommended in particular Harlow Shapley, the vigorous director of the Harvard Observatory in Massachusetts, and wrote a reference letter to him putting in a strong word. Shapley and his PhD advisor, Henry Norris Russell at Princeton, were then to American astronomy what Eddington was to British astronomy; Shapley had gotten his start at the famed Mount Wilson observatory in California where Edwin Hubble would work, and he had succeeded Pickering at the Harvard Observatory which was trying to catch up to Mount Wilson (Science would run in the Shapley family’s blood: Harlow’s son Lloyd won the 2012 Nobel Prize in Economics for his work on game theory). Shapley and Eddington collectively gave Cecilia an opportunity she wouldn’t have gotten otherwise. Another critical early mentor was a Cambridge instructor named J. W. Lowrie, a talented observational astronomer who had lost a leg during the Great War and who spent countless hours and sleepless nights teaching Cecilia how to scour the sky looking for different kinds of stars and recording their types and positions.
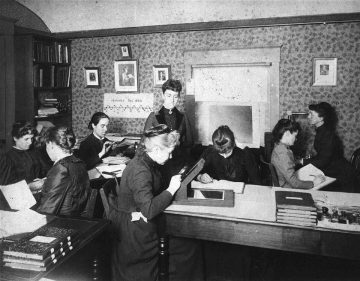
Armed with her father’s violin case, Payne came to America in the early 1920s to a place that was later to become legendary for its success – all led by women – in charting the fates and properties of hundreds of thousands of stars. Edward Pickering who was the first director of the Harvard Observatory had hired women “computers” in the 1890s to do the grunt work of tedious astrophotography. These women would use the 15-inch telescope located a few miles away from the Harvard campus to photograph the light coming from different stars and classify them. Later many of these women were to become famous, foremost among them Henrietta Leavitt whose discovery of a relationship between the luminosity and period of variable Cepheid stars was to pave the way toward Edwin Hubble’s discovery of the expansion of the universe.
Leavitt had died a few years before Payne got there, but others like Annie Cannon and Antonia Maury were still doing important work. Payne joined this cadre of women led by a man. It was an unusually egalitarian environment, with Shapley giving the women considerable freedom in their work and organizing informal “Shapley Squares”, meetings where an invited visitor would hold forth on a piece of science with everyone sitting in a square and brainstorming. It was at one of these meetings that Cecilia met Henry Norris Russell who was the leading American astronomer of his time. Russell would become another mentor to Cecilia. He was immediately impressed, not just by Cecilia’s drive but by her substantial knowledge of languages and the classics that rivaled his own interests in these subjects. He quickly became a mentor to her.
Russell, Eddington, Shapley and the grand old men of astronomy had a vision of stars that was rooted in conservatism, especially when it came to their composition. The path to figuring out what the stars are made of started in the mid 19th century when two German chemists, Gustav Kirchhoff and Robert Bunsen (of Bunsen burner fame) found that they could correlate the wavelength of different lines when light from a source was broken up into its constituents to the elemental composition of the source. Spectra could be both emission spectra and absorption spectra, produced by elements when they either emitted or absorbed radiation. When I was in high school I remember how we studied the vivid changes in color in – ironically – a Bunsen burner flame when a wire dipped in a strontium or copper salt was held in it; strontium gave a deep red, copper a cool blue. Spectroscopy was an epochal discovery that suddenly gave scientists a way to figure out what the heavens are made up of from the comfort of their earthly laboratories; comparing the emission spectra of earthly elements with the absorption spectra of stellar elements can point to the presence of the same element in both environments. Using Kirchhoff and Bunsen’s new technique, in 1868 the British astronomer Norman Lockyer discovered a faint yellow line in the sun’s spectra that corresponded to the element helium; helium thus became the first terrestrial element to be discovered in the stars. From this time onwards spectroscopy became scientists’ favorite tool for probing the structure of both terrestrial and extraterrestrial matter.
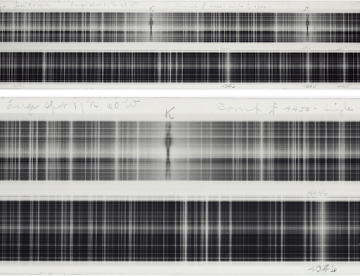
By the time Payne started studying physics, spectroscopy had been responsible for some of the greatest discoveries of the previous few decades. Most notably, it was the spectra of hydrogen that had inspired Niels Bohr to come up with his famous model of the atom; when Bohr found that his model explained the wavelengths emitted in these spectra, he knew he was on the right track. Continuing to try to explain the spectra of higher elements would lead to the discovery of quantum mechanics in the 1920s. Astronomers had also found that the spectral lines from starlight could tell you how hot the star was, since different temperatures produced different lines. It was Annie Jump Cannon who had created the now-standard classification of stars based on the spectra-temperature relationship. The astronomers at Harvard had made hundreds of thousands of spectrographic plates based on directing the light from distant stars through a telescope into a camera. The photographic plates were often messy and could contain hundreds of different lines; sorting through these and reaching a conclusion about the temperature and composition of the stars was like cutting through a thick jungle to ascertain what part of the world it might be located in. The process needed acute powers of observations, an outstanding appetite for tedious work and infinite patience. The women at Harvard all had these qualities, but the one quality they lacked was Payne’s Cambridge-earned combination of knowledge of atomic physics and astronomy.
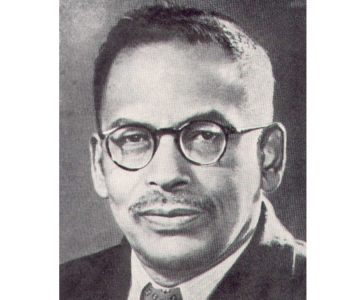
Because of her background in spectroscopy, Payne knew that she could not only relate the lines in the stellar spectra to the temperature of the stars but also tease them apart to uncover the true composition of a star. The key to unlocking that relationship had been discovered by an Indian physicist named Meghnad Saha who had grown up in a poor family in Bangladesh and had once been expelled from his school for taking part in the Indian independence movement. Saha’s ionization equation related the ionization state of an element to its spectral signature and the conditions inside the star in which that element existed. Ionization takes place when there is enough energy for an electron to be knocked out from the orbit of an atom; at extremely high temperatures, a fully ionized state of matter called plasma exists, and at intermediate temperatures there is a mix of ionized and unionized atoms. Like Kirchhoff and Bunsen’s spectroscopy, Saha’s formula gives earthly astronomers a tool to find out what’s happening inside stars. The key to interpreting the spectra was to realize that an element and its ionized form would result in different spectral lines.
The difficulty came from the fact that it was exceedingly hard to distinguish an ionized form of an element from a different element in the thicket of spectral lines. Until then the variety of spectral lines in a star’s spectrum had been attributed to separate elements. Not just this, but the elemental composition displayed by the spectra was thought to resemble that found on the earth. This ‘principle of uniformity’ had been a pillar of astronomical belief, and Eddington, Shapley and Russell all subscribed to it. Curiously enough, their attitude was not different from some of the ancients who believed the heavens to be uniform and undisturbed by sudden breaks or violent phenomena. But when Payne teased the various spectral lines apart and applied Saha’s formula to them, she realized that rather than corresponding to different elements, many of the spectral lines corresponded to ionized and unionized states of a single element – hydrogen. A smaller but still substantial number corresponded to helium. And she did not have access to just some data for reaching this groundbreaking conclusion; thanks to the efforts of her predecessors, she could analyze thousands of spectra from thousands of different stars. Each data point said the same thing – the stars are made mostly out of hydrogen, with a little bit of helium thrown in along with a very tiny percentage of elements like lithium and oxygen, what astronomers called “metals”. This composition was clearly completely different from the elemental composition of the earth where oxygen and silicon are the first and second-most abundant elements. The principle of uniformity had been dealt a shattering blow.
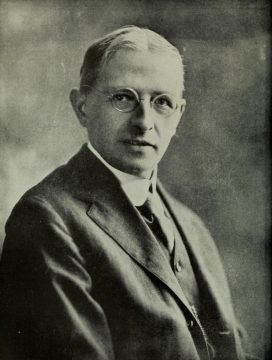
Payne’s discovery came in 1925. At that point Shapley was lobbying Harvard to establish a separate astronomy department and have Payne’s work become the first PhD thesis from that department. The advocacy was in Shapley’s interest because he wanted the world to see his observatory as an independent institution and not just as an offshoot of the Harvard physics department. When Payne wrote her work up as a PhD thesis, Shapley sent it to his former teacher Henry Norris Russell whose blessing would be critical in getting it accepted. This is when everything ran into a snag. Russell wrote back to Payne saying that he liked and respected her work but was sure that her conclusion about the preponderance of hydrogen in stars and the universe was a mistake. Perhaps the data itself was wrong, or perhaps the mathematics that she was using was wrong. Russell was polite but insistent that she modify her conclusion in some way. At this point Payne was at a crossroads; she could dig in and defend her results or she could accept Russell’s misgivings, modify her conclusion and receive her PhD.
She chose the latter path, but not without leaving a trapdoor in her story, one that made it clear especially in retrospect that she wholeheartedly believed in her results. “Although hydrogen and helium are manifestly very abundant in stellar atmospheres”, she wrote, “the actual values derived from the estimates of marginal appearance are regarded as spurious.” This statement made it clear that while the conclusions may be wrong, she was still the one who had made the discovery. Posterity has judged Payne’s PhD thesis titled “Stellar Atmospheres” to be possibly the most important PhD thesis in the history of astronomy. When her thesis was submitted, the department thought it was so obviously important that they didn’t realize they had to officially tell her that she had passed; a colleague gave a despondent and weeping Cecilia the good news. But whatever the fate of her thesis, at that point the grand old men of astronomy whose cherished principle of uniformity a twenty-five-year-old had just laid to rest could lay easy themselves. After submitting her thesis Payne left for England and met Eddington. That she still stood up for her beliefs was obvious when she clearly told him that she had surmised that the stars were made up of hydrogen: “Surely you don’t mean it’s in the stars but rather around the stars, don’t you?” was Eddington’s response. It was a specious bit of reasoning, but for the time being it served to deflect from Payne’s troubling observations.
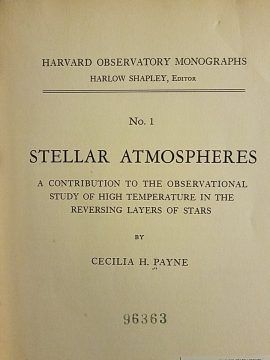
What contributed to the reaction to Payne’s results? It wasn’t sexism alone; Russell, Shapley and Eddington all genuinely admired Payne’s scientific talents and had consistently encouraged her, even if they reflected some of the skepticism and patronizing attitudes about female scientists emblematic of their times. There were at least a few bonafide scientific reasons which legitimately thwarted acceptance of the conclusion: Saha’s equation wasn’t that well tested, as he himself admitted, and hydrogen’s light atomic weight might exaggerate its appearance in stellar spectra. The science of divining the details of stellar composition was still in its infancy, and the confident certainty of posterity presented itself as the haze of uncertainty then. But it’s probably true that a determined twenty-five-year old man – perhaps one from a family of Boston Brahmins – would at least have been allowed to mount a more aggressive defense of his work.
At twenty-five Cecilia had accomplished the most important discovery of her career. Even if one discounted the seminal findings about hydrogen, her other work became nationally and internationally known and she was asked to give talks at a variety of scientific conferences at home and abroad. In 1933, just as the ominous clouds of Nazism were appearing on the horizon, a young Russian eagerly strode up to her at a conference in Germany and thrust a handful of papers he had written into her hands. Well-built and an ardent communist studying in Germany which was then the capital of the scientific world, Sergei Gaposchkin had seen the writing on the wall. Seeing the Nazis’ oppression of communists and wanting to work in the United States, he asked Cecilia if she could help him get a job at the Harvard Observatory, essentially a ticket for escaping obliteration. Some strange bond must have connected the two, because Gaposchkin was far less well known than Payne and she had never heard of him before. Perhaps she saw a kindred existence in the hardscrabble manner in which Gaposchkin had persevered to learn astronomy in the decrepit scientific facilities in the Soviet Union, perseverance that might have reminded her of her own struggles in Cambridge. Whatever the reason, in a few months she had convinced Shapley to invite Gaposchkin for a fellowship at the observatory. Three months later they were married.
Sadly the relationship was not one of intellectual equals. Sergei was a reasonably good but not exceptional astronomer and worked hard at first, and he and Cecilia studied the variable stars whose study Henrietta Leavitt had pioneered. But he soon became testy, growing bitter when Shapley gently asked him not to publish mediocre or incomplete work. Matters were not helped when Sergei who was a bodybuilder used to flex his muscles and flirt with some of the female staff. But the couple’s early years were happy and Cecilia gave birth to three children, two boys and a girl. Not only did she raise them well as a somewhat strict and austere mother but she lectured at conferences while pregnant, setting a model example for other women to follow before even Shapley had to sheepishly tell her to stay home during her third pregnancy because he thought too many eyebrows were being raised. In spite of Sergei and Cecilia’s relationship troubles and her somewhat aloof and science-obsessed personality, all three of her children became accomplished: her sons got degrees in geophysics and astrophysics from MIT and Harvard and her daughter graduated from Swarthmore and became a technical writer at the Smithsonian.
But Payne’s reputation still did not guarantee success in the establishment. When Shapley retired she would have been his natural successor. The job went instead to Donald Menzel, a former student of Henry Norris Russell. Menzel did write an official letter to Payne’s eleven-year-old son prohibiting him from running around in the observatory, but more importantly he noticed that Payne’s salary was still paltry compared to bonafide professors, and he not only doubled her salary but petitioned Harvard to make her a full professor. In 1956, Payne thus became the first female full professor picked from within the Harvard faculty, and a few years later became the first woman to head a department there. She had reached the pinnacle of success on a mountain whose foundations had been laid by illustrious women predecessors. Payne continued to lead the department into the 1970s, trying to balance research with teaching and administrative duties. She mentored many successful students including Frank Drake who is a leader in the SETI (Search for Extraterrestrial Intelligence) community. Sadly, her lifelong habit of chain smoking finally caught up to her – the habit had been picked up growing up in prudish Edwardian England as an act of defiance – and she died of lung cancer in 1979.
Cecilia Payne Gaposchkin left an extraordinary legacy, both scientific and human. Her discovery that the sun and stars were made up of hydrogen led directly to efforts to figure out the source of the sun’s energy. The culmination of these developments was Hans Bethe’s 1938 discovery of the so-called proton-proton cycle that fuels the nuclear reactions keeping the sun burning; he won a well-deserved Nobel Prize in 1965 for his discovery. Interestingly, Bethe who became a grand old man of physics and a pillar of conscience for the scientific community had to be reminded in a 1999 interview that it was Payne who had discovered the hydrogen whose identity as a nuclear fuel he had figured out. Another grand old man, Cecilia’s mentor Henry Norris Russell, finally admitted in 1929 that her thesis had been correct. Payne’s PhD thesis set a standard for both women in science and for PhD theses – she joins a select few people like John Nash and Frank Wilczek who published groundbreaking work while in graduate school – and it propelled many women to go into astronomy, including Richard Feynman’s sister Joan Feynman. Cecilia never won a Nobel Prize for her discovery and never seems to have been nominated, attesting to the late recognition of her significance. The other underappreciated character in this story is Meghnad Saha: he was nominated seven times, including twice by the well known American physicist Arthur Compton, but he too did not win. Ultimately of course, while accolades are important, Saha and Payne-Gaposchkin’s work speaks for itself.
The tools of spectroscopy that Cecilia used to make her famous discovery grew into a spectacular scientific discipline and sent tentacles into fields Cecilia couldn’t even have dreamt of. It has revolutionized not just physics and astrophysics but fields as disparate as biochemistry and materials science. In recent years, the spectra of light reflected from the surface of faraway worlds has revealed the astonishing abundance of exoplanets, a field that was recognized the Nobel Prize last year. In various incarnations – optical, microwave, infrared, gamma – spectroscopy has allowed astronomers to probe the grandest scales of space and time, reaching to the birth of the universe itself. It was Saha’s formula that in part enabled scientists to calculate the temperature and time at which protons and electrons combined to form neutral hydrogen after the Big Bang, and it was this event that allowed the universe to become opaque to radiation that then continued undisturbed for 13.5 billion years until it was discovered by two physicists working on a little hill in New Jersey in 1964.
Cecilia stands as a role model for women in science in myriad ways. She always struck out on her own and sheer doggedness marked her efforts from beginning to end. But it should also be remembered that science is teamwork, and it takes a village to make even a very gifted scientist successful. The people in Cecilia’s village were her parents who spared no expense in indulging her interests in science and nature and bringing her up the same as any son. They were Frances Gray and the women in England who thought that women should make careers for themselves. They were the women of Harvard Observatory who blazed the trail and collected the hundreds of thousands of data points that made her discovery possible. They were the lesser-known and better-known men of physics and astronomy – Eddington, Rutherford, Shapley, Lowrie, Menzel – who at least partly overcame their own biases to see something exceptional in Cecilia and encouraged her astronomical explorations. And finally it was the process of science itself, that restless endeavor which moves men and women’s inner spirit and inexorably propels them forward, that made Cecilia strive constantly to look up toward the stars and find out what the cosmos is made up of. The lesson she left us, both from her life and her discoveries, is an enduring one: we exist in the fleeting intervals between the stars, but as brief as these moments are, it’s up to us to make them meaningful.